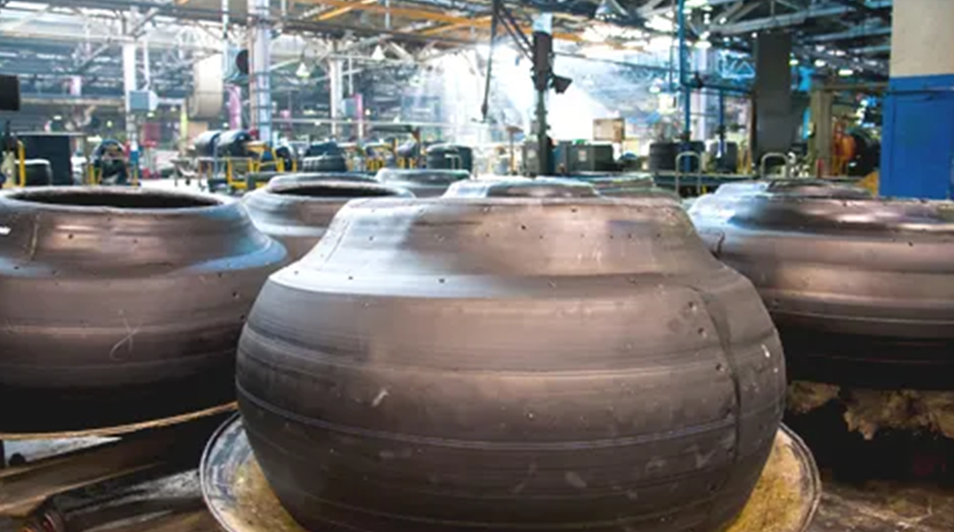
Uses Of Liquid Ring Pumps In Plastics, Rubbers & Composites
In the industrial realm, the significance of liquid ring pumps in processing plastics, rubbers, and composites cannot be overstated. To understand their utility, it’s intriguing to delve into the ancient processes that shaped our planet.
Picture this: Prehistoric algae and zooplankton, once thriving in ancient waters, met their demise. Their organic remains gracefully descended to the waterbeds of oceans, lakes, ponds, and rivers. Over time, layers of silt and sand meticulously veiled their bodies. The accumulating silt, under mounting pressure, metamorphosed into sedimentary rock, encapsulating the remnants.
Enter anaerobic bacteria, catalysts of transformation. They meticulously broke down sulfates and nitrates within the organic matter, yielding hydrogen sulfide and paving the way for a remarkable alchemical process. Gradually, under the dual forces of time and pressure, the bacteria themselves succumbed. What remained were the elemental building blocks: hydrogen and carbon derived from the once-vibrant algae and zooplankton.
This is where the contemporary narrative intersects with ancient chemistry. Liquid ring pumps, with their versatile applications, come into play. In the intricate processes of plastics, rubbers, and composites manufacturing, these pumps prove indispensable. Their ability to handle diverse fluids, including those derived from the decomposition of ancient life forms, underscores their importance in modern industrial setups.
As we navigate the complex landscape of industrial processes, the role of liquid ring pumps becomes clear—a crucial component in the synthesis of materials that shape our present and future.
View our Products
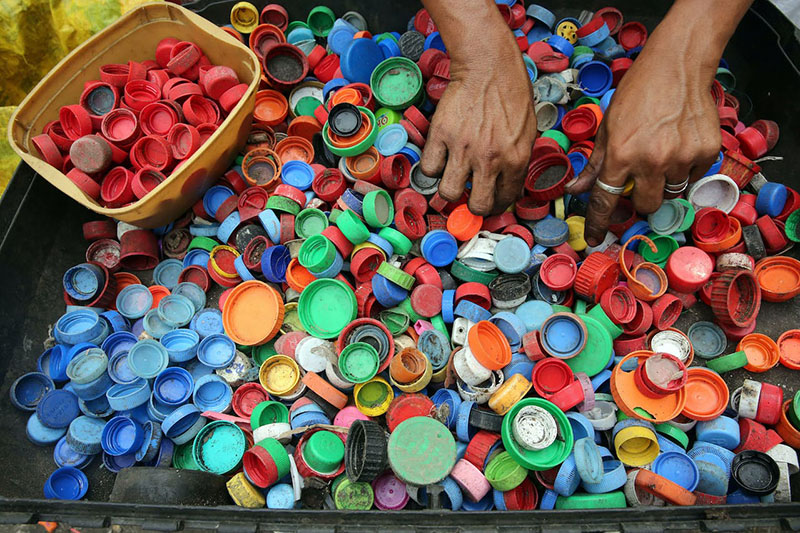
At the right time and under the right pressure, these kerogens can be removed as crude oil or gas by drilling down to the layer of earth where the kerogen is. The oil is pumped out and sent to refineries.
The oil is fed into a furnace, evaporates, and is led to a large fractional distillation column at a specific height above the bottom, where a temperature gradient is applied. The lighter gasses rise to the top and the components with the highest boiling point remain as a liquid at the bottom. The multi-layered distillation column separates each component and condenses them separately. Among these components are naphtha (an oil that is a mix of paraffin and alkylbenzenes) and ethane. These undergo steam cracking, or pyrolysis, to create short, usually unsaturated (only carbon-carbon single bonds) hydrocarbon monomers suitable for polymerization.
As the name implies, heat in the form of steam is used to decompose (lysis), or “crack”, the larger hydrocarbons into smaller constituents in an oxygen-deprived furnace (to prevent oxidation of potential hydrocarbons). These cracking furnaces must be tailored to the chemical composition of the feedstock to ensure the proper breakdown; a result of hydrogen gas and carbon atoms separating would be a waste of time and resources. The yield is quenched, leaving short hydrocarbon monomers called olefins that include ethylene and propylene, two key ingredients in polymerization.
There are two kinds of polymerization: chain-growth polymerization (addition reaction) and step-growth polymerization (condensation reaction). In the addition reaction, only one type of monomer is used. The monomers are combined and form double or triple carbon bonds. This method does not involve any loss of molecules. In the condensation reaction, atoms like nitrogen, oxygen, and hydrogen are combined with different kinds of monomers. These different things combine and leave a condensate. This reaction does involve the loss of molecules, and the condensate is usually water.
The result of the polymerization of propylene and ethylene is plastic, of which numerous kinds have unique qualities. These hydrocarbons are inert and very hard to degrade, so they are the preferred choices for plastic, synthetic rubber and many composites. After polymerization, the plastic is ready to be formed.
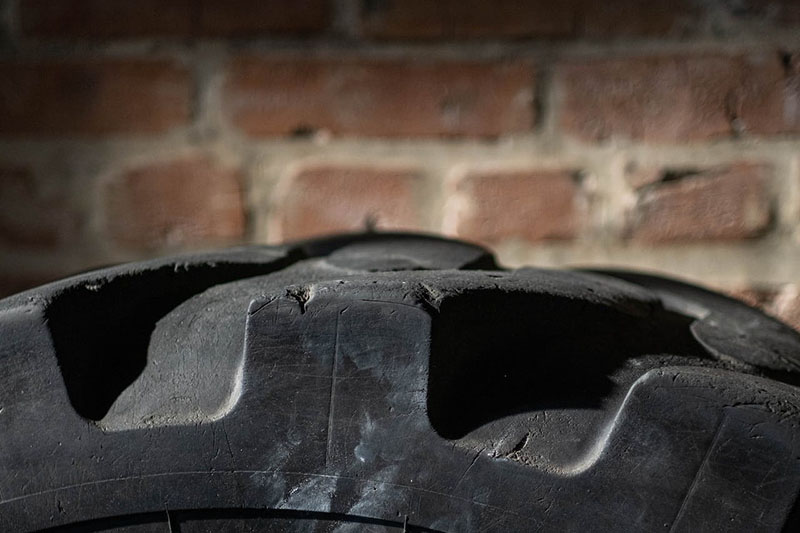
Once the polymer is formed, it is available for use. Of the methods of forming plastic, there is 3D printing, CNC machining, polymer casting, rotational molding, vacuum forming/thermoforming, injection molding, extrusion, and blow molding. The decision of which to use depends on lead time, budget, design complexity, and production volume.
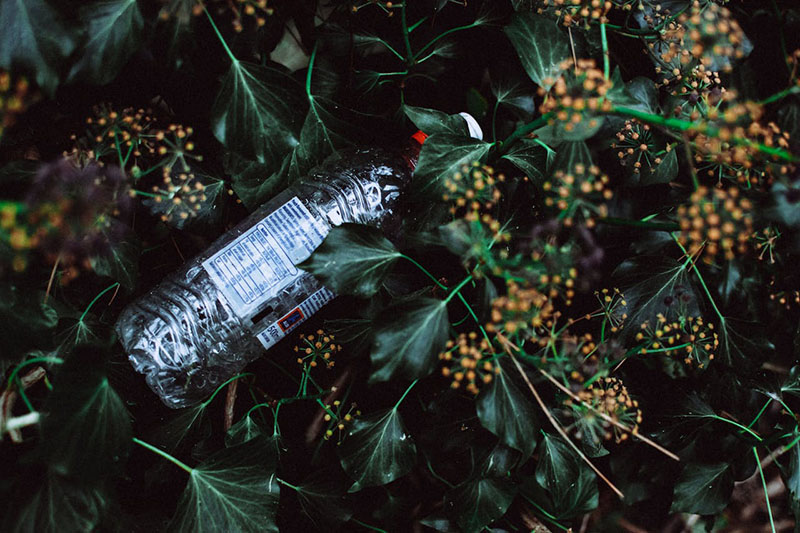
Vacuum forming involves heating a plastic sheet over a 3D mold and using suction to pull it over the mold. The vacuum cools the plastic by a small amount while pulling it on the mold. The plastic is cooled further and trimmed. Vacuum forming can get expensive for the extremely high volume of product production. Still, it is suitable for thousands of applications, such as car door liners, dental aligners, boat hulls, utensils, garden equipment, and bathtubs. The design can get quite intricate, but has its limits, only being able to suction thin sheets. This makes for narrow walls and relatively simple geometries compared to 3D printing.
Applications of Liquid Ring Vacuum Pumps
Liquid ring vacuum pumps emerge as indispensable tools in the realm of industrial processes, particularly in the intricate domains of plastics, rubbers, and composites manufacturing.
In the context of vacuum distillation of crude oil, liquid ring vacuum pumps exhibit their prowess. By establishing a distillation column under sub-atmospheric conditions, these pumps reduce the heat energy required for evaporation. This is achieved by lowering the boiling point of each component through vacuum pressure. Notably, mixtures that may have formed azeotropes under atmospheric pressure can be efficiently separated with a variance in pressure, enhancing susceptibility to phase shift.
The complexity of this process involves handling various materials, including dirty substances like asphalt, saturated vapors such as paraffin fumes, and combustible gases like methane. To optimize efficiency, pumps involved in the process must constantly handle and pump the separated elements. Liquid ring vacuum pumps excel in this role, creating a deep vacuum that prevents azeotropic mixtures and lowers the boiling point of each crude oil component. Their constant vacuum ensures efficient operation, surpassing pumps with strokes.
Moreover, these pumps exhibit resilience in the face of challenges. Trapped dirt, sludge, or suspended particles do not impede their performance; they pass through seamlessly. The pumps also condense condensable vapors, further boosting pumping efficiency. The choice of metal for pump construction allows for corrosion resistance and explosion-proof capabilities, accommodating volatile substances like methane, butane, and diesel with minimal risk.
Liquid ring vacuum pumps extend their utility to vacuum forming processes. Their design, consisting of a few simple parts, facilitates adaptation to a wide array of applications. This simplicity not only streamlines maintenance but also contributes to robustness and longevity. These pumps operate with minimal energy requirements, devoid of the need for lubrication or oil sealing, resulting in low installation and operation costs. Importantly, the continuous suction capability remains uninterrupted, even in the presence of solids or liquid slugs, ensuring seamless performance in scenarios like vacuum forming heated plastic.
In summary, the uses of liquid ring pumps in plastics, rubbers, and composites extend far beyond conventional applications, demonstrating their versatility and efficiency across diverse industrial processes.